Research at Jefferson Laboratory
The Heavy Photon Search
The Heavy Photon Search (HPS) is a new experiment at Jefferson Laboraty that will search for the "heavy photon" (a.ka."dark photon" or A'). This heavy photon is predicted by many of the current extensions of the Standard Model and could account for dark matter annihilations.
The HPS was approved by PAC37 with the condition of a successful test run. We hope to stage this test run and take data in 2012, before the Jlab shutdown and subsequent upgrade to 12 GeV. The main experiment will run in the 12 GeV era. To read more and get the full proposal go to the Heavy Photon Search (HPS) page.
An additional search to the HPS experiment will that for "true muonium", a bound state of a muon and anti-muon. Such a state is very rare and has never been observed before.
Silicon Vertex Tracker for CLAS12
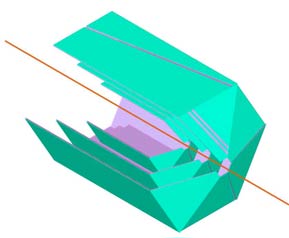
Simulated picture of the SVT detector, part of the CLAS12 detector.
The UNH Nuclear Group just started a new project for the CLAS12 detector. The accelerator at Jefferson Lab will be upgraded in a few years to deliver an electron beam with an energy of 12 GeV, and a new spectrometer is being built for Hall B to make use of the upgraded accelerator. The innermost detector system of the upgraded CLAS detector system is a Silicon Vertex Tracker (SVT), which is made of three consecutive barrels consisting of silicon microstrip detectors, as can be seen in the simulated picture of the SVT to the right. These detectors will provide tracking capabilities for charged particles produced in the target. We are constructing a testing facility for the SVT detectors in the Nuclear Lab at UNH. The testing facility will involve a complete detector readout system and an x-y-z positioning table, which will position an infrared laser over the silicon detector to be tested. The silicon strip detectors will be tested for quality assurance and to evaluate their performance before their installation in the CLAS12 detector system.
Software Development for CLAS and CLAS12
Spin Structure Studies
Over 99% of the known mass of the universe is composed of two particles: the proton and the neutron. Collectively known as the nucleon, they are the basic building blocks of all atomic nuclei. However, these `basic' particles themselves possess a complex substructure. Our understanding of this structure has benefited greatly from a program of electron-nucleon scattering spanning many decades, which indicates that the nucleon consists of point-like quarks and gluons. The latter serves as mediators of the strong interaction that holds the quarks together, and it is this force which is ultimately responsible for nuclear binding. One of the driving goals of nuclear physics is to characterize the fundamental properties of the nucleon to allow comparison with theory. Only then can we obtain a quantitative understanding of the nucleon's substructure, ultimately providing a description of atomic nuclei from first principles.
Nucleon spin structure is characterized by two dimensionless functions known as g1 and g2. Precise measurements of both these quantities are needed for a complete understanding of spin-dependent properties. UNH NPG members are primarily interested in reactions where the momentum transfer Q2 of the electron scattering process is small. The wavelength of the electro-magnetic probe is inversely proportional to the momentum transfer, so when Q2 is small, the full volume of the nucleon is probed and the interactions among constituents can not be ignored or treated perturbatively. In this complex `long distance' region, the nucleon spin structure functions (SSF) become notoriously difficult to derive from first principles. However, insight has been gained by testing fundamental sum rule predictions which govern the behavior of their moments. Some of these, such as the Bjorken sum rule, provide direct tests of Quantum Chromodynamics, while others such as the extended GDH, and the Burkhardt-Cottingham (BC) sum rules allow us to test some of the underlying assumptions inherent in the theory. As one of the fundamental spin observables of the nucleon, precise knowledge of the SSF at low Q2 has broad ranging impact, and is needed for disciplines as diverse as Lattice Gauge calculations to atomic physics.
E08-027: A Precise Measurement of the Proton's g2 Structure Function at Low Q2
NPG member Karl Slifer serves as spokesman for E08-027, which is scheduled to run in 2011 in Hall A at Thomas Jefferson National Accelerator Facility. This experiment will provide the first precise measurement of the proton's g2 structure function at low Q2. These data will address several interesting open questions. For example, existing neutron data supports the BC sum rule, while previous proton measurements were found to be inconsistent with the sum rule prediction. Even more compelling, it was found that state-of-the-art chiral perturbation theory calculations exhibit a significant discrepancy with the longitudinal-transverse polarizability δLTn. Measuring the proton polarizability is a crucial step in resolving this puzzle. Finally, lack of knowledge of the g2p structure function at low Q2 is one of the leading uncertainties in hydrogen hyperfine splitting calculations.
EG4: The Extended GDH Sum at Low Q2
Karl Slifer is also a co-spokesperson of E06-017, which is part of the EG4 experimental rungroup in Jefferson Lab's Hall B. EG4 measured the proton and deuteron spin structure function g1 at low Q2. The results of this measurement will allow a test of chiral perturbation theory calculations at low Q2. UNH Research Associate Sarah Phillips recently began to analyze the deuteron quasielastic data with an ultimate goal of providing the first high quality deuteron polarized cross sections and asymmetries in this region.
Color Transparency
Search for the Phi-- Pentaquark
DVCS with CLAS and CLAS12
Research with Neutrons
NPDGamma

The NPDGamma Experiment.
The NPDGamma experiment is designed to measure or put a severe upper limit on the contribution of the one of the four known fundamental interactions, the weak interaction, to the process of the capture of a (very) slow neutron by a single proton, which is dominated by the strong interaction. The process results in the emission of a single photon (or gamma ray) of 2.22 MeV. In the experiment, the incoming beam of slow neutrons is polarized, i.e. the neutrons, which possess intrinsic spin, all have their spins aligned in the same direction. If there is a contribution from the weak interaction, there will be a very slight difference in the number of gammas emitted to the left or right relative to the incoming beam. If the orientation of the spins is reversed, the left-right gamma asymmetry will also be reversed. By flipping the spin back and forth, this asymmetry can be determined to high precision and the strength of the weak contribution can be obtained and compared to predictions from the Standard Model which incorporates the weak and strong interactions. The experiment will be carried out using neutrons in the Fundamental Neutron Physics Beamline (FnPB) at the Spallation Neutron Source (SNS) at Oak Ridge National Lab.
ABba
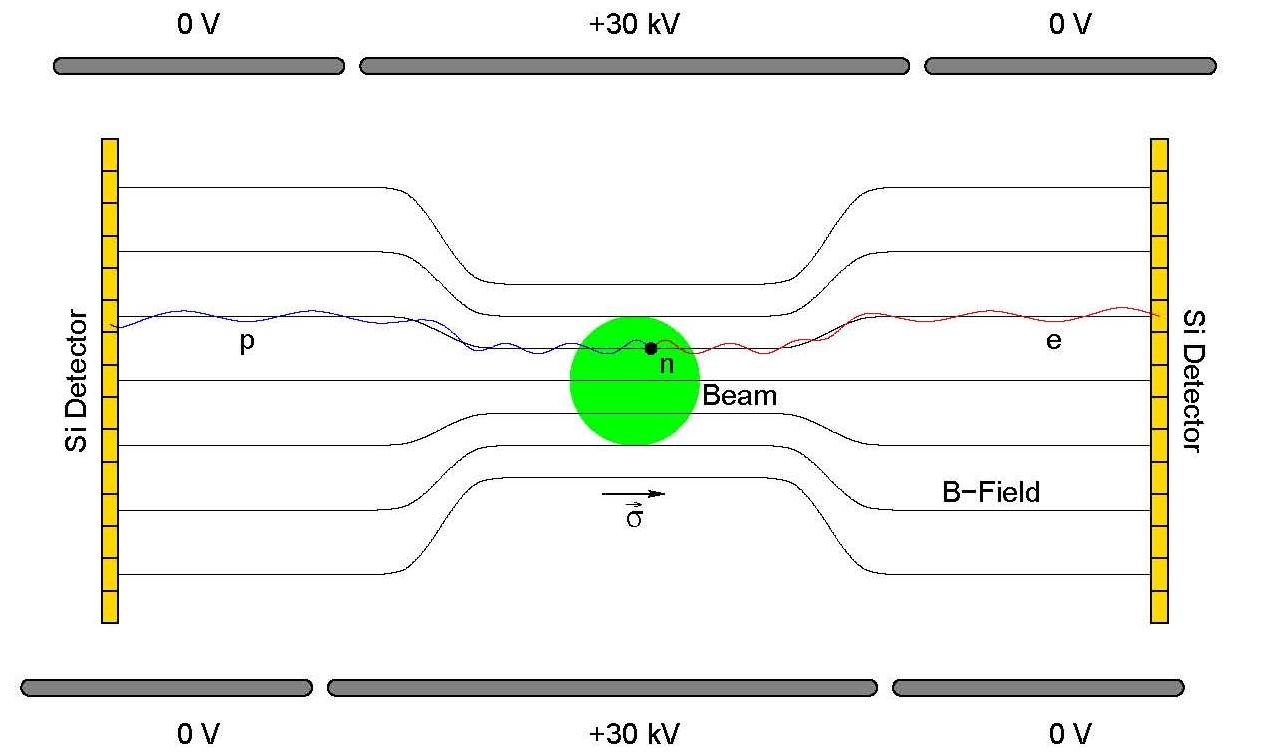
A diagram of the conceptual design for the abBA spectrometer.
The ABba experiment is another experiment aimed at testing the Standard Model. It is a precision measurement of 4 angular coefficients, standardly identified as A, B, a, and b, which describe the directional probabilities of emission of the electron, proton, and antineutrino relative to each other and to the the the direction of the neutron spin in the decay process: n --> e + p + antineutrino, the fundamental neutron beta decay. In fact, we know that the Standard Model (SM) is wrong and that these coefficients must deviate from the SM prediction at some level of precision. A measurement of that deviation will point to the new physics beyond the SM. How do we know the SM is wrong? The SM is a model based on neutrinos (and antineutrinos) having zero mass, but recent measurements have shown that these particles are not massless, although we don't know exactly what that non-zero mass is. This experiment will also be carried out at the FnPB at the SNS once NPDGamma is completed, by observing the directions of the e and p from the decay of spin oriented neutrons as they travel through the detection apparatus. The direction of the antineutrino and its energy can be inferred from the e and p by conservation of energy and momentum.
HIGS
The High Intensity Gamma Source (HIGS) being developed at Duke University will provide a unique beam of medium energy gamma rays: mono-energetic to high precision and 100% polarized. We plan to use this beam to make accurate measurements of the distributions of charge and magnetism within the neutron and proton as well as the spatial and spin distributions of the constituent quarks by using this 100% polarized photon beam on highly spin oriented hydrogen, deuterium, and 3He targets. The latter two will provide information on spin oriented neutrons.
BLAST
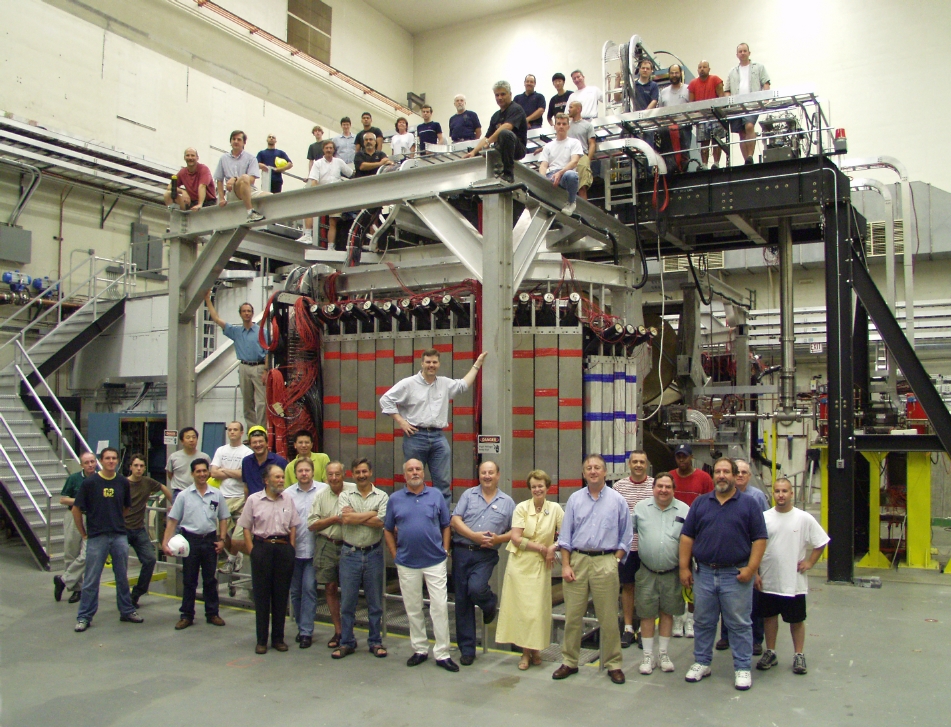
The BLAST Collaboration and Spectrometer at MIT-Bates.
The BLAST (Bates Large Acceptance Spectrometer Toroid) detector was developed by a collaboration centered at the Bates Accelerator Facility at MIT in which UNH played a prominent role. During its operation at Bates, it was used to study the scattering and reactions of high energy spin oriented electrons from targets of spin oriented hydrogen and deuterium. From these data, we have been able to unravel the charge and magnetization distributions of the proton, deuteron, and neutron. The work has resulted in 11 Ph.D.s, 3 of which were earned by UNH graduate students. Now BLAST is slated for a reincarnation. It will be moved from Bates to DESY in Hamburg, Germany where it will be used to compare the scattering of electrons and positrons on the proton. Recent experiments at Jefferson Lab in Virginia have demonstrated a marked difference in the inference of the charge and magnetization distributions of the proton when using two radically different techniques which were theoretically expected to yield the same results. The leading candidate for the explanation of this difference also predicts a difference in positron and electron scattering rates, which would be expected to be the same to a first approximation. The DORIS storage ring at DESY provides the world's highest intensity positron beam as well as an intense electron beam.